![]() |
Retos Terrícolas
Earth Science Blog | Blog sobre Geociencia
2025-04-25
Before multiplying CO2 emissions by 100, global warming was already in the news:
2025-04-24
The largest flood in Earth’s history burst through Gibraltar and Sicily and refilled the entire Mediterranean in just a few years
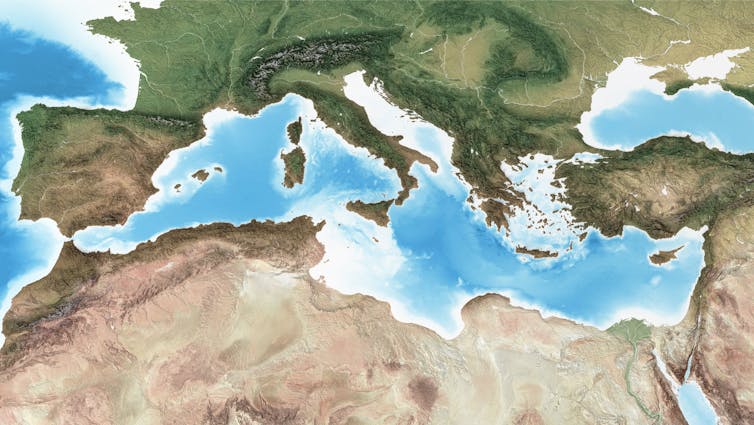
A little over 5 million years ago, water from the Atlantic Ocean found a way through the present-day Strait of Gibraltar. According to this theory, oceanic water rushed faster than a speeding car down a kilometre-high slope towards the empty Mediterranean Sea, excavating a skyscraper-deep trough on its way.
The Med was, at the time, a largely dry and salty basin, but so much water poured in that it filled up in just a couple of years – maybe even just a few months. At its peak, the flood discharged about 1,000 times the water of the modern-day Amazon river.
At least, that’s the thesis one of us put forward in a 2009 study of an underwater canyon excavated along the Strait of Gibraltar, which he presumed to have been carved out by this massive flood. If correct, (and some scientists do dispute the theory), the so-called Zanclean megaflood would be the largest single flood recorded on Earth.
But extraordinary claims like this require extraordinarily solid evidence. Our latest research investigates sedimentary rock from the Zanclean era that seems to record how the water surged through a gap between modern-day Sicily and mainland Africa to refill the eastern half of the Mediterranean.
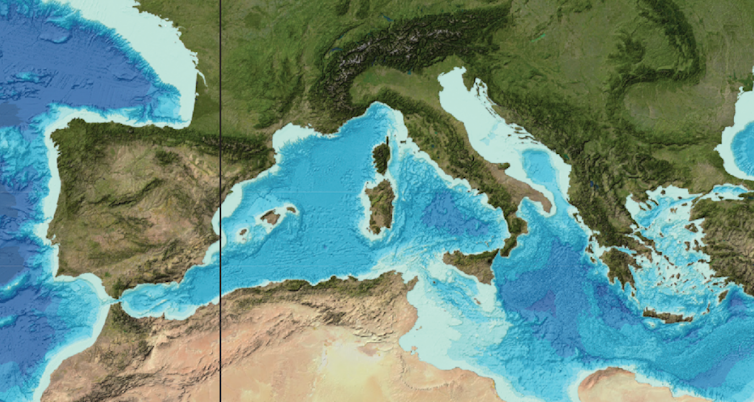
How scientists tracked down the megaflood
Our finding is the latest twist in a story that began in the late 19th century. That’s when geologists studying salt-rich rock outcrops around the Mediterranean became increasingly aware that something unusual had happened between roughly 5 and 6 million years ago, well before the glaciations of recent ice ages: the sea had dried up. They named that age “Messinian” and the drying up eventually became known as the Messinian salinity crisis.
In the 1970s, scientists for the first time drilled deep below the Mediterranean into sedimentary rocks from the Messinian age. They made three surprising discoveries. First, they found a massive layer of salt – kilometres thick – below much of the seafloor. This confirmed that a vast environmental change had happened about 6 million years ago, just when tectonic plates shifted and the sea became largely isolated from the Atlantic Ocean.
Second, right above this salt layer, they found sediment with fossils from shallow, low-salt lakes. This suggested that the Mediterranean Sea dropped to more than a kilometre below today’s level, and as most of the water evaporated, salt was left behind. A series of lakes would have remained in the lowest parts of the basin, refreshed and kept relatively salt-free by streams. This interpretation was also supported by seismic surveys of the seabed which revealed rivers once cut through a dry landscape.
And third, the rocky layers above the salt abruptly shifted back to more typical deep sea sediment. (We now know that less than 11% of Mediterranean marine species survived the crisis, showing just how big and lasting the impact was on life in the sea). The term Zanclean Flood was coined in the 1970s to refer to the end of the crisis, without scientists really knowing what it consisted of or the timescale taken to refill the dry Mediterranean basin.
A cataclysmic refill
The next breakthrough came in 2009, when geophysical data for the planned Africa-Europe tunnel through Gibraltar suggested that a huge underwater trench between the Atlantic Ocean and the Mediterranean Sea must have been created by a sudden and cataclysmic flood.
Our latest research backs up this hypothesis. As part of a team led by Maltese seabed scientist Aaron Micallef, we explored the region where the flood water filling the western basin of the Mediterranean should have run into a ridge of higher land connecting modern-day Africa and Italy, known as the Sicily Sill. Was there any evidence, we wondered, of a second megaflood as the eastern Mediterranean filled up?
Piecing together the puzzle
Giovanni Barreca, one of our co-authors on the recent paper, grew up in southern Sicily. He long ago realised that the low hills near the coast are an extension of the Sicily Sill over which the megaflood must have progressed from west to east. The area, he thought, might contain clues.
Our team visited this part of Sicily and noticed that the hills were indeed unusual. Their aligned and streamlined shapes separated by deeply eroded depressions are very similar to streamlined hills in Washington state in the US. Those Washington hills were carved out by a megaflood at the end of the last Ice Age when the vast Lake Missoula dammed up behind a glacier and emptied catastrophically.
If those hills and depressions in Sicily were also shaped by a huge flood, then rock debris eroded from the base of the depressions should be found dumped on top of the hills, more than 5 million years later.
Sure enough, we did find jumbled and contorted rock debris up to boulder size along the crest of the hills. They were the same types of rock found within the depressions as well as further inland.
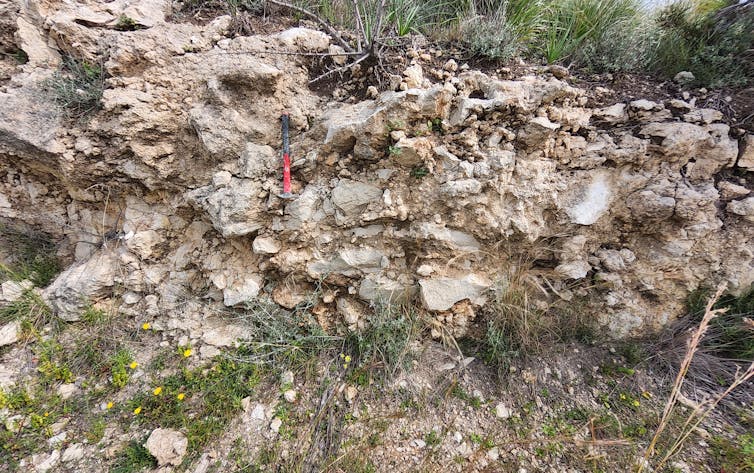
To double check our work, we developed a computer simulation (or “model”) of how flood waters might have crossed one part of the Sicily Sill. It showed that the flood flow would indeed mimic the direction of the streamlined hills.
In fact, the model showed that the hills would have been carved out by water 40 metres or more deep, travelling at 115 kilometres per hour (71mph). In the one area we modelled, 13 million cubic metres of water per second would have flooded into the eastern Mediterranean basin (for reference: the Amazon today is about 200,000 cubic metres per second). Remarkably, this is still only a fraction of the water that first flowed through Gibraltar and then into the eastern Mediterranean basin near Sicily.
Don’t have time to read about climate change as much as you’d like?
Get a weekly roundup in your inbox instead. Every Wednesday, The Conversation’s environment editor writes Imagine, a short email that goes a little deeper into just one climate issue. Join the 45,000+ readers who’ve subscribed so far.
Daniel García-Castellanos, Earth scientist, Instituto de Geociencias de Barcelona (Geo3Bcn – CSIC) y Paul Carling, Emeritus Professor of Geomorphology, University of Southampton
This article is republished from The Conversation under a Creative Commons license. Read the original article.
¿Cómo acabar con el 89% de las especies marinas del Mediterráneo? Lecciones del pasado

¿Cómo acabar con el 89 % de las especies marinas del Mediterráneo? Lecciones del pasado
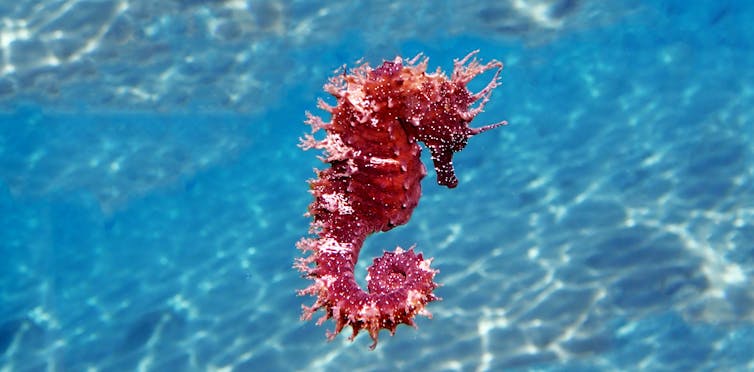
¿Qué pasaría si convirtiéramos todo el mar Mediterráneo en una gigantesca salina? ¿Sobreviviría su fauna? ¿Cuánto tiempo necesitaríamos para recuperarla? Parecerían preguntas intrascendentes si no fuera porque un arquitecto bávaro dedicó buena parte de su vida a ese proyecto: construir una gran presa a través del estrecho de Gibraltar y dejar que el Mediterráneo se secara para colonizar el terreno ganado al mar.
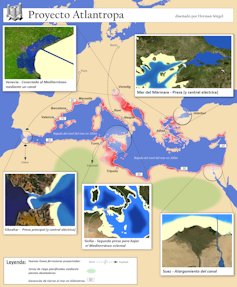
Herman Sörgel organizó conferencias y documentales y recaudó financiación hasta los años 50 para un proyecto que, pensó, fomentaría la cooperación entre África y Europa y electrificaría ambos continentes con gigantescos proyectos hidroeléctricos.
Lo que Sörgel no sabía es que su sueño ya había sido realidad cinco millones y medio de años antes, a finales de la era del Mioceno, sin más proyecto detrás que el de las leyes naturales.
Cuando el Mediterráneo se secó
Desde la década de los 70, varias generaciones de geólogos y geofísicos marinos han confirmado la existencia de una capa de sal de entre uno y tres kilómetros de espesor enterrada en la mayor parte del Mediterráneo más profundo.
Se trata de casi un millón de kilómetros cúbicos de sal que atestiguan un breve periodo de aislamiento del Mediterráneo del resto del océano. Breve en el sentido geológico, porque el episodio duró unos 190 000 años. Y no solo eso: el seco clima mediterráneo provocó la evaporación de sus aguas y expuso a la intemperie gran parte de su suelo marino tras la precipitación de toda aquella sal.
El responsable no fue ningún excéntrico arquitecto alemán sino la tectónica de placas. La cuenca mediterránea, atrapada entre dos continentes que continúan hoy aproximándose hasta dos centímetros cada año, quedó aislada del Atlántico y sus aguas se evaporaron rápidamente debido al clima árido que domina nuestro planeta en estas latitudes.
Este escenario, conocido como la crisis de salinidad del Messiniense (el último periodo del Mioceno), es el mayor cataclismo sufrido por la Tierra desde la caída del meteorito que acabó con los dinosaurios no voladores y con la era Mesozoica hace 65 millones de años.
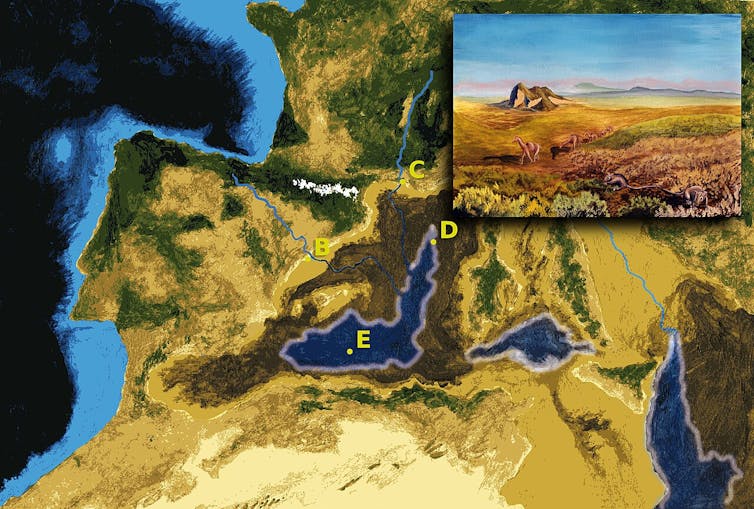
Gracias a ello, no es necesario ningún experimento geoingenieril para responder a la pregunta inicial: ¿cómo de resiliente es la vida marina frente a una crisis medioambiental de este calibre?
La respuesta acaba de ser publicada en la revista Science, en un estudio liderado por Konstantina Agiadi, de la Universidad de Vienna, con la colaboración del CSIC y de 25 paleontólogos de 25 institutos europeos. Tras reunir toda la información fósil del Mediterráneo de entre hace 11 y 2 millones de años, nuestros resultados sugieren que la vida marina autóctona fue prácticamente extinguida durante el aislamiento del Mediterráneo y que la posterior recolonización por especies atlánticas dio origen a la fauna mediterránea tal y como la conocemos hoy en día.
Las especies autóctonas, las desaparecidas y las inmigrantes
Analizando estadísticamente la información de más de 750 artículos científicos hemos podido documentar 22 932 muestras de vida marina fósil que documentan 4 897 especies mediterráneas antes de la salinización del Mediterráneo. De las 779 especies posiblemente endémicas (encontradas solo en el Mediterráneo), solo 86 seguían presentes después del fenómeno salino.
A modo de ejemplo, todos los corales tropicales que abundaban en el Mediterráneo antes del gigantesco cambio medioambiental desaparecieron. En cambio, alguna especie de sardina aparentemente endémica logró sobrevivir. Un ejemplo de mamífero superviviente es el sirenio, emparentado con los actuales manatíes y dugongos (también conocidos como vacas marinas).
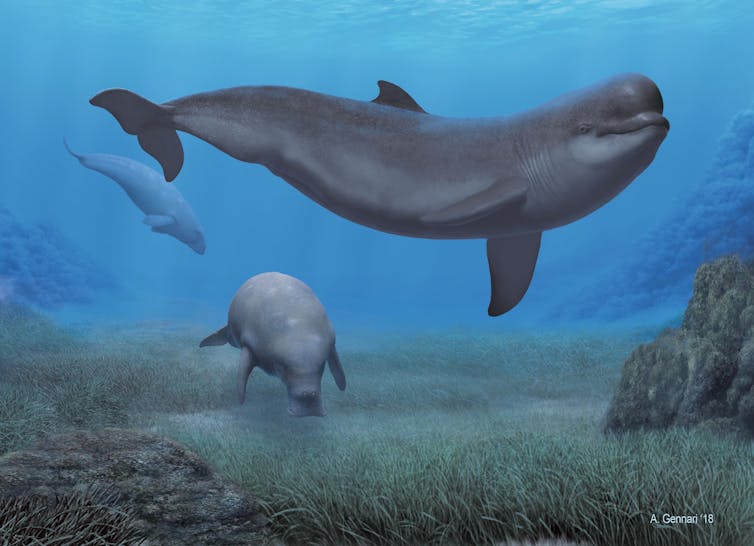
Debido a lo limitado y fragmentado del registro fósil, no podemos asegurar que estas especies fueran todas endémicas ni que no hubieran sobrevivido fuera del Mediterráneo, de ahí el valor de realizar este estudio de forma estadística con un gran número de especies. Pero las que lo eran, ¿dónde consiguieron sobrevivir? ¿Qué refugios encontraron para evitar el radical aumento de salinidad y temperatura?
Estas preguntas siguen sin respuesta, pero sí hemos podido constatar que los cambios de las poblaciones son debidos al reemplazo por especies atlánticas tras la reinundación, más que a una rápida adaptación al nuevo medio hipersalino. Es decir, la vida no tuvo tiempo para adaptarse y las especies extintas fueron sustituidas por otras exóticas, atlánticas.
Algunas especies icónicas como el gran tiburón blanco y el delfín aparecieron por primera vez en el Mediterráneo solo tras la crisis. Y aún más interesante: la actual mayor riqueza faunística del Mediterráneo occidental se estableció tras la reinundación, mientras que anteriormente el número de especies era mayor en el Mediterráneo oriental (mar Jónico y mar de Levante).
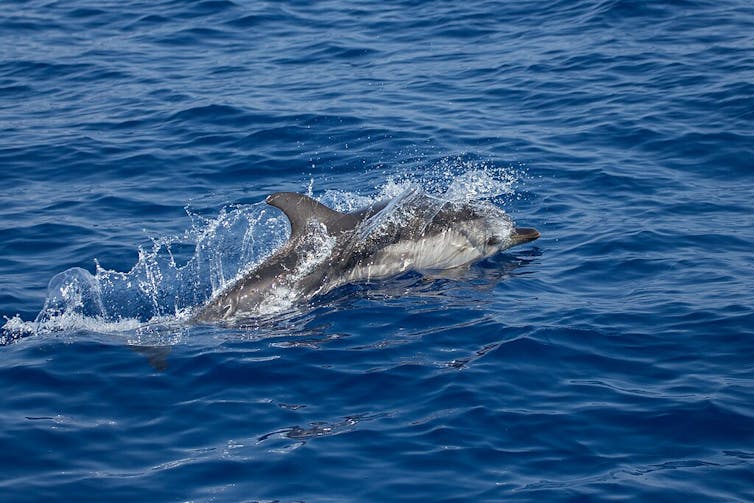
Lecciones sobre las extinciones masivas
El impacto del aislamiento del Mediterráneo sobre su fauna y flora fue enorme, destruyendo la mayoría de sus ecosistemas y su conectividad. Otro importante resultado que hemos obtenido estudiando aquel gigantesco experimento natural es que la recuperación en términos de número de especies duró más de 1,7 millones de años. Esta lenta recuperación de la riqueza de los ecosistemas mediterráneos proporciona la primera cuantificación detallada de la respuesta biológica estadística a un evento de extinción de esta magnitud.
La biodiversidad mediterránea actual es muy alta gracias a la presencia de numerosas especies endémicas. Nuestros resultados sugieren que esto también era así hace 6 millones de años, pero que la gran mayoría de esas especies endémicas desaparecieron durante el aislamiento y salinización del Mediterráneo.
Y quizá otra lección aprendida de este estudio sea que, por muy tentadores proyectos de geoingeniería que se nos ocurran y nos permitan soñar con mantener el actual ritmo de emisiones y de destrucción de ecosistemas, vale la pena aprovechar bien las experiencias que nos brinda el pasado geológico de la Tierra antes que experimentar con ella. El Mediterráneo, pese a mantener el océano global como reservorio de especies, tardó millones de años en recuperarse. Nadie sabe aún cuánto tardará en recuperarse la vida marina de un cambio a escala global como el que está en curso.
Daniel García-Castellanos, Earth scientist, Instituto de Geociencias de Barcelona (Geo3Bcn – CSIC)
Este artículo fue publicado originalmente en The Conversation. Lea el original.

La pista del metano para encontrar vida en el subsuelo de Marte
Marte podría ser habitable. Su superficie resulta inhóspita para cualquier organismo terrestre, por sus bajas temperaturas y humedades y las excesivas radiaciones nocivas que recibe, así que la búsqueda de vida en el Planeta Rojo se centra en el subsuelo.
La Tierra alberga microorganismos que proliferan en los intersticios y fisuras de las rocas, hasta a varios kilómetros de profundidad. ¿No podría ocurrir lo mismo en Marte? Y entonces, ¿cómo lo encontramos?
Aquí entra en juego el metano, la pista que hemos seguido para dar con un lugar marciano idóneo, que podría resultar habitable.
Según nuestro estudio, la región de Acidalia Planitia, en el hemisferio Norte de Marte, es la que reúne las condiciones menos adversas para que microorganismos productores de metano (metanógenos) similares a los terrestres pudieran proliferar bajo su superficie.
La huella biológica
El metano es una molécula clave en el estudio del origen de la vida en la Tierra y de supuestas señales de vida fuera de ella. En nuestro planeta, lo producen organismos unicelulares procariotas del reino de las arqueas, llamados metanógenos. Estos microorganismos proliferan en todos los ambientes sin oxígeno (anóxicos) pero con disponibilidad de fuentes de energía como moléculas orgánicas simples, hidrógeno molecular o dióxido de carbono (CO₂).
La presencia de metano se interpreta como una posible huella de la actividad biológica. Pero este hidrocarburo también se puede generar abióticamente (sin intervenir seres vivos). Por ejemplo, a partir del hidrógeno molecular que se produce por hidratación de rocas silícicas profundas como la peridotita en contacto con agua (un fenómeno común conocido como serpentinización). Entonces haber encontrado metano en Marte no significa necesariamente haber encontrado señales de vida.
Del metano de Marte se habla desde la década de 1960 a partir de una detección errónea, y cada nueva detección ha encendido largos debates que han avivado la intriga: ¿podría el subsuelo de Marte albergar seres metanógenos tal como los que conocemos en la Tierra? ¿Cuenta con las mínimas condiciones ambientales y los recursos necesarios para su proliferación? ¿Donde habría que buscarlos?
Habitats potenciales
En nuestra investigación abordamos estas preguntas recopilando la información de organismos metanógenos en la subsuperficie terrestre. Tras cruzar sus hábitats con la información disponible de la geología de Marte, hemos podido identificar regiones con mayor probabilidad de albergar vida de estas características.
En la Tierra existen tres hábitats subterráneos que podrían ser análogos del subsuelo marciano:
Pequeñas masas de aguas hipersalinas submarinas localizadas principalmente al fondo del Mediterráneo y del Mar Rojo. El agua con sal disuelta permanece líquida a temperaturas inferiores a los -20⁰ C, permitiendo la proliferación de metanógenos a temperaturas “marcianas”.
Lagos subglaciales, abundantes sobre todo en la Antártida, y que podrían tener análogos bajo el hielo del polo Norte de Marte.
Masas de agua que fluyen en fracturas y poros de las rocas continentales profundas. Este es el escenario más probable. La litosfera superior de Marte es muy porosa y fracturada debido en parte al impacto de meteoritos, y cuenta con la presencia de elementos radiactivos como el Uranio. Esto permite que la temperatura aumente rápidamente con la profundidad y facilita la producción de hidrógeno molecular, el recurso energético por antonomasia de los organismos metanógenos terrestres, a partir del agua.
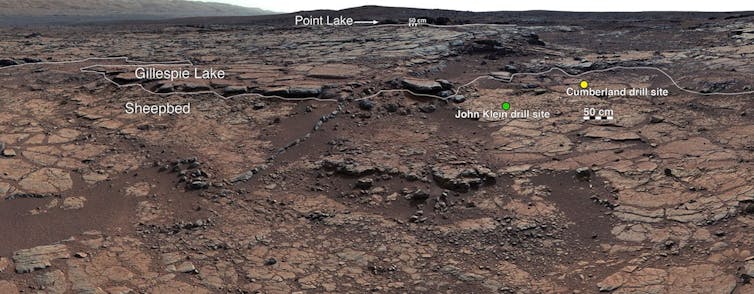
La ecología importa
Nuestro análisis evidencia que los metanógenos se encuentran regularmente en estos tres hábitats, pero escasean a temperaturas inferiores a los cero grados Celsius. También se detectan con más dificultad en las fracturas de rocas profundas, aunque los requisitos mínimos para su proliferación se cumplen.
Esto induce a pensar que sería más difícil encontrarlos, en caso de que estuvieran, en un hábitat de rocas profundas fracturadas de Marte.
Otro aspecto crucial es que, en los hábitats mencionados anteriormente, son minoritarios respecto a las bacterias y dependen y compiten con ellas. Así pues, si estamos dispuestos a imaginar unos supuestos metanógenos en la subsuperficie marciana parecidos a los terrestres, también allí deberían asociarse a otros organismos para formar ecosistemas complejos.
La región de Acidalia Planitia como el lugar más favorable
Finalmente, a partir de la literatura científica, hemos analizado la distribución de elementos radioactivos en la superficie de Marte y tenido en cuenta su contribución a la energía geotérmica del planeta.
También hemos considerado la distribución de agua en el subsuelo, así como la temperatura media superficial.
Teniendo en cuenta la presencia de sales y de porosidad del subsuelo, deducidos a partir de datos de la misión InSight de la NASA, concluimos que la región de Acidalia Planitia, en las tierras bajas del hemisferio norte marciano, es la que reúne las condiciones menos adversas para que organismos metanógenos similares a los terrestres pudieran proliferar bajo la superficie, a una profundidad de entre 4 y 9 km.
Nuestro estudio no es el primero que apunta a esta región para buscar vida en Marte: la zona se caracteriza por la presencia de centenares de pequeñas estructuras cónicas recientes que recuerdan a los volcanes de lodo terrestres y que podrían reflejar movimientos no tan antiguos de agua líquida hasta la superficie.
Esta confluencia de distintas técnicas refuerza la conjetura de que Acidalia Planitia podría ser una región prometedora (o la menos descorazonadora) en la búsqueda de vida fuera de la Tierra.
Andrea Butturini, Profesor agregado. Biogeoquímica y ecologia microbiana de ambientes extremos, Universitat de Barcelona; Daniel García-Castellanos, Earth scientist, Instituto de Geociencias de Barcelona (Geo3Bcn – CSIC); Octavi Fors Aldrich, Investigador en Astrofísica, Universitat de Barcelona y Robert Benaiges-Fernández, Investigador, Microbiólogo, Bioinformático
Este artículo fue publicado originalmente en The Conversation. Lea el original.
2025-03-29
2025-03-07
Del planeta Tierra a los exoplanetas: nuevas fronteras de la investigación sobre la vida en astrobiogeología
Foto: NASA
¿Es potencialmente habitable el exoplaneta Kepler-452 b, localizado a 1.400 años luz de la Tierra? ¿En qué otros puntos del Universo se ha podido desarrollar el fenómeno de la vida? ¿Cuál sería la bioquímica más plausible para la vida extraterrestre? Todas estas incógnitas sobre la investigación de la vida dentro y fuera del sistema solar son el hilo conductor del viaje narrativo que emprende el libro (In)habitabilidad planetaria. Fundamentos de astrobiogeología (Marcombo), una obra coordinada por Andrea Butturini, profesor del Departamento de Biología Evolutiva, Ecología y Ciencias Ambientales de la Facultad de Biología de la UB.
En el libro, que recuerda la relevancia de incorporar el fenómeno de la vida a la visión científica del Universo, también participan los expertos Carme Jordi, de la Facultad de Física y el Instituto de Ciencias del Cosmos (ICCUB) de la UB y del Instituto de Estudios Espaciales de Cataluña (IEEC); Jordi Urmeneta, de la Facultad de Biología y el Instituto de Investigación de la Biodiversidad (IRBio) de la UB; Ignasi Ribas, del Instituto de Estudios Espaciales de Cataluña (IEEC) y el Instituto de Ciencias del Espacio (ICE, CSIC), y Daniel García Castellanos, del Instituto de Geociencias de Barcelona (GEO3BCN-CSIC).
Entre la Tierra y las estrellas: en busca de vida ahí afuera
Con una perspectiva interdisciplinaria, el libro subraya el valor de la astrobiogeología como punto de encuentro de múltiples disciplinas que invitan tanto al debate sobre paradigmas aceptados como al discurso de carácter más especulativo. «Es un campo de investigación que tiene un crecimiento enorme. Es obvio que su desarrollo depende de la tecnología y de grandes inversiones al alcance de pocas entidades y países, los cuales a menudo forman consorcios para optimizar recursos», apunta Andrea Butturini, profesor del Departamento de Biología Evolutiva, Ecología y Ciencias Ambientales de la UB.
«El aspecto más crucial de la astrobiogeología —continúa el experto— es que se nutre de la interacción entre la física, la química, la geología y la biología, convergencia que está potenciando el desarrollo de trabajos científicos en los que se implican investigadores de todo el mundo no necesariamente vinculados a las instituciones de investigación en ciencias del espacio. A menudo, esa literatura científica tiene un fuerte componente especulativo —muy atractivo desde un punto de vista académico y didáctico— que sería muy interesante introducir en los estudios universitarios, y de paso, con ello, promover aún más la unión entre todas estas disciplinas».
¿Estamos solos en el Universo?: una revolución en la visión del cosmos
Como explica Ignasi Ribas, director del IEEC e investigador del ICE, «establecer el contexto en que se desarrolla la vida en el Universo es uno de los grandes retos de la ciencia para las próximas décadas». Con el fin de alcanzar ese objetivo, resulta imprescindible un enfoque pluridisciplinar, ya que los numerosos factores que intervienen en ello comprenden áreas de conocimiento tradicionalmente separadas.
«Esta aproximación integral que combina la astrofísica, la biología y la geología —todas ellas llevadas a sus límites— deberá permitirnos responder una de las grandes preguntas, no solo de la ciencia sino de la humanidad como un todo: ¿estamos solos en el Universo? La trascendencia de la respuesta implicará una nueva revolución en todos los aspectos», subraya Ribas.
En ese viaje intrépido del conocimiento humano por el cosmos, los expertos buscan «las señales que la existencia de vida en un exoplaneta deja en su atmósfera», explica Carme Jordi, catedrática del Departamento de Física Cuántica y Astrofísica de la Facultad de Física de la UB. Y puntualiza: «Para averiguar la composición química y la estructura térmica de las atmósferas utilizamos la espectroscopia, y tomamos medidas en el momento en que el planeta transita por delante de una estrella».
El estudio de las atmósferas exoplanetarias mediante la espectroscopia infrarroja es uno de los objetivos científicos del futuro telescopio espacial James Webb, de la NASA y la ESA. «Además, la próxima misión ARIEL, de la ESA, es la primera dedicada exclusivamente al mismo objetivo, y también para una amplia muestra de exoplanetas, que en este caso se estudiarán con espectroscopia óptica y del infrarrojo cercano», añade la investigadora, también miembro del ICCUB, el IEEC y el equipo científico de la misión Gaia, de la ESA.
Geología de la Tierra y de los exoplanetas
Para estudiar y modelizar los procesos geológicos más allá de la Tierra, «aplicamos todo lo que hemos aprendido sobre nuestro planeta», apunta Daniel García Castellanos, experto del ICTJA-CSIC. La exploración in situ de otros mundos y la proliferación de descubrimientos de planetas fuera de nuestro sistema solar son una excelente oportunidad para contrastar lo que creemos saber sobre el sistema de la Tierra, los procesos que se repiten fuera y la frecuencia con que lo hacen.
García Castellanos menciona algunos ejemplos al respecto: «La erosión producida por el agua líquida ha sido esencial para regular el clima terrestre de forma que pueda albergar vida, ¿pero significa eso que sin un ciclo del agua similar al nuestro no puede haber vida? También sabemos que el campo magnético terrestre ha impedido la pérdida de volátiles de nuestra atmósfera, incluida el agua. ¿Podríamos predecir, con lo aprendido en la Tierra, si un lejano exoplaneta tiene su campo magnético bien desarrollado, antes de verlo?». Por otra parte, el modelado o la simulación de esos procesos permite predecir mejor los escenarios que se encontraría una posible misión en un planeta o una luna del sistema solar.
Materia viva: tras la pista de la vida extrema en el cosmos
El estudio de los microorganismos que viven en ambientes extremos ha cambiado de forma drástica la forma de evaluar si es posible la presencia de vida en otros lugares del espacio. En este sentido, el libro abre horizontes a la investigación sobre la vida en el cosmos y ofrece escenarios alternativos de las posibles biosferas alienígenas.
«Se ha podido constatar que la vida es muy tenaz y que en la Tierra aparece en todos aquellos lugares donde realmente es posible, incluso en aquellos donde, por sus condiciones aparentemente inhóspitas, parecía imposible. Eso nos ha llevado a pensar que los ambientes extraterrestres donde habría podido desarrollarse la vida son muchos más de los que se creía hasta hace poco», explica Jordi Urmeneta, profesor del Departamento de Genética, Microbiología y Estadística de la Facultad de Biología y miembro del Instituto de Investigación de la Biodiversidad (IRBio).
Como invitación directa al pensamiento más especulativo, el libro deja margen a la duda en un contexto en que el objeto de estudio de la astrobiogeología —la vida extraterrestre— brilla por su ausencia. «Quizás el día en que se detecte su existencia, la astrogeobiología entrará en una fase más ortodoxa, y la faceta especulativa perderá relevancia», postulan finalmente los autores.